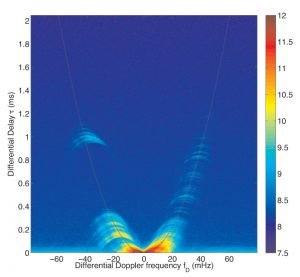
The result of isolating the unique radiation “signatures” for each individual pair of interfering speckles. Credit: J-P Macquart.
With an average density of less than 1 proton per cubic centimetre, the matter that exists between stars might seem unimportant. But in actual fact, our galaxy the Milky Way and the stars, planets and people it contains wouldn’t be here without it.
Comprised mostly of gas (~99%) and dust (< 1%), the Interstellar Medium (ISM) is described by astronomers and astrophysicists as ‘clumpy’, meaning the material has a tendency to coalesce through gravitational forces and collisions.
Throughout the ISM, massive stars, planetary nebulae and supernova explosions drive strong winds and shock waves through the medium. These mechanisms deposit energy, sustain the highly inhomogeneous state of the ISM and enrich it with heavy elements from which the next generation of stars and planets will eventually form.
In recent years evidence has accumulated pointing to the existence of ultra-compact structures within the ISM, the properties of which require explanations well beyond the scope of current models. As these incredibly dense objects pass through our line of sight to a quasar or other compact radio source, they trigger Extreme Scattering Events (ESEs) resulting in large increases in the radiation received from sources beyond the object.
Most current explanations posed to explain the dense object responsible for ESEs are problematic or controversial. Several require increasing the known density of objects a thousand times for structures 0.3-1 AU in size, and dictate that they should be so over-pressured that they would simply explode. But if this were the case, these objects would be quite rare; whereas astronomical observations have shown them to be fairly common.
Until now the major obstacle to resolving these shortcomings has stemmed from a lack of observations, but recent progress made by radio astronomers has led to the first ever image of ‘speckles’ in the Interstellar Medium.
Shine a laser along a rough surface, and a circle- shaped speckle pattern will appear around the beam, corresponding to points of constructive interference between the reflected wavefronts. In the laboratory environment, information about this speckle pattern can be used to measure the properties of a reflecting surface down to scales approaching 1μm. But to apply this technique on an astronomical scale a naturally occurring cosmic laser is called for, which is where a class of objects known as ‘pulsars’ comes to the rescue.
Pulsars are highly magnetised, rotating neutron stars that emit a highly coherent beam of electromagnetic radiation. These objects are so compact that the radio-wavelength radiation emitted behaves much like an interstellar laser. But when it comes to imaging and making sense of a source heavily scattered by the turbulence of the ISM, the task is not for the fainted hearted.
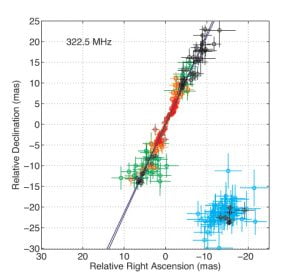
The speckle image of Pulsar PSR B0834+06, produced using this new technique. Credit J-P Macquart.
The breakthrough to achieving this scientific feat was in realising that it is possible to isolate the radiation pattern for each individual pair of interfering speckles, or “sub-images” of the pulsar. Each pair of interfering sub-images creates an unique “signature” that changes over time and encodes information relating to the exact location of the speckles. By recording the intensity fluctuations, in both time and frequency, as the pulsar moves relative to the Interstellar Medium it is possible to generate a secondary spectrum like the one shown below.
However, this in itself is not enough to form an image.
Having isolated the radiation from each interfering pair, the next step is to determine which part of the scattering disk the radiation has come from. This is achieved by performing Very Long Baseline Interferometry (VLBI), to observe the fluctuations in the scintillation pattern, using a number of radio telescopes separated by several thousand kilometres.
So far this ground-breaking technique has been pioneered by a collaboration of radio astronomers from the National Radio Astronomy Observatory, the University of California, the University of Massachusetts and the International Centre for Radio Astronomy Research in Western Australia. Using the Green Bank Telescope and the Arecibo Observatory, the largest steerable and non- steerable radio dishes in the world respectively, the results of these early efforts has revealed for the first time the turbulent nature of interstellar space over a large range of scales, from 1.6 billion km down to 4.5 million km (0.03 of the distance between the Earth and the Sun).
Armed with this new technique for imaging the turbulent Interstellar Medium on tiny scales, well below 1011m, the prospects for understanding the strange nature of interstellar structures is bright.
Author: Dr Jean-Pierre Macquart